
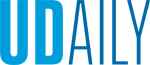
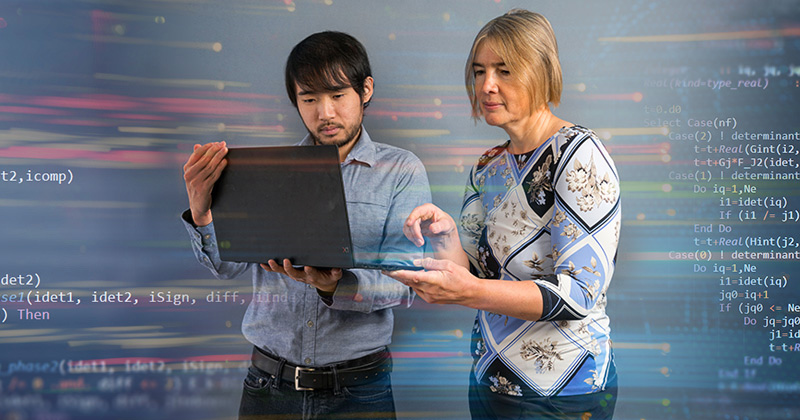
Cosmic code
Photo illustration by Jeffrey C. Chase March 02, 2023
UD physicist, postdoctoral researcher help solve 40-year-old astrophysics puzzle
Scientists are constantly searching for ways to understand how galaxies, black holes and stars form, grow and reorganize in the universe. Astrophysics is the field tasked with helping to explain some of these things.
A lot of what astronomers and physicists know comes from analyzing the light that we receive from these cosmic phenomena using theoretical calculations, only some of which can be validated experimentally. This causes tension between what theoretical calculations suggest and what experimental data can prove.
One current example of this involves a 40-year-old puzzle in astrophysics where experimental observations and theoretical predictions of two specific radiation lines of iron ions found in space plasmas disagreed by up to 15%, a difference several times larger than expected. The controversy cast doubt on how well scientists understand and describe very hot gases that originate from cosmic X-rays.
X-rays are a form of energetic radiation emitted by very hot gases in space that exist in the vicinity of objects, such as black holes, huge clusters of galaxies, in-stellar flares and even the corona of the sun. Data about the intensity of these X-rays are important for understanding physical conditions in space, such as temperature and density.
University of Delaware theoretical physicist Marianna Safronova and postdoctoral researcher Charles Cheung are part of an international team led by the Max Planck Institute for Nuclear Physics that recently resolved this longstanding disagreement using an extremely precise experiment. Improved theoretical calculations contributed by Safronova and Cheung played an important role in the work.
Thinking outside the lines
Plasmas are very hot gases that make up about 99% of all visible matter in space. Because they are so hot, the atoms inside these gases break into their components, leaving positively charged ions and electrons that move independently.
Safronova is an expert in computing the properties of atoms. Atoms have distinct levels of energy, and when an electron jumps from one energy state to another, it emits a particle of light called a photon. How fast this jump takes place is called the transition rate, which changes depending on the energy levels that are involved in the process.
Analyzing this light is the main way astronomers learn about stars and galaxies in the cosmos. But these experimentalists need help from theoretical physicists like Safronova, who can calculate where to look for these transitions. To do this, theorists use atomic-scale models and then point experimentalists in the right direction.
Safronova explained that in the problem they were working on, a theoretical calculation of a key property of two very bright, visible transitions of iron called 3C and 3D (which occur in most hot astrophysical plasmas) strongly disagreed with experimental results. The property in question showed how strong one transition was relative to the other.
The discrepancy had previously been attributed to theory not being accurate enough. Yet, no matter how theoretical physicists like Safronova modified the advanced calculations, the predicted transition rate didn’t change in any meaningful way. This led the theorists to remain certain their calculations were correct, but it still bothered them.
So, Safronova and her collaborators at other institutions worked to improve their methods for calculating this transition rate using supercomputers, including UD’s Caviness and DARWIN high-performance parallel computing systems. Key to this process was Charles Cheung, a postdoctoral researcher in Safronova’s group who earned both bachelor’s and doctoral degrees in physics at UD in 2016 and 2021. Cheung is credited with writing the new parallel version of the atomic code that helped solve this long-standing discrepancy.
“Charles was instrumental in terms of theory for making this work happen,” said Safronova.
Cheung recalled the laborious process of running code on a single computer for an earlier paper and waiting weeks for the computer to return a single number, then repeating the calculation over and over with different parameters. The power of high-performance parallel computing, harnessed by Cheung’s new parallel code, greatly speeded up this process on the current work.
“For that first paper it took me months of runtime just to figure out the numbers I needed. Now, I can rerun that original two-week calculation and have an answer in 15 minutes,” said Cheung, the paper’s second author. “I can run problems that are over 100 times larger, too, to explore exciting new systems in atomic physics that are of extreme interest to experimental groups for building quantum sensors.”
The new version of the code allowed the theoretical team to make much larger calculations than previously possible while reducing numerical errors. Cheung’s first version of parallel code scaled with about 50% efficiency, but with additional refinements, today main parts of the code perform with 99 to 100% efficiency.
“With our new code, we were able to put uncertainty numbers on our predictions,” he continued. According to Safronova, this ability to put precision on theoretical predictions in such complicated systems is new, and it provided even greater confidence that the team’s atomic-scale models were correct.
Meanwhile, Safronova’s experimentalist collaborators decided to redo the experiment at PETRA III, a German synchrotron light facility at the DESY laboratory outside Hamburg. The improved experimental precision allowed the research team to obtain data on the tails of the spectral lines they observed. The new measurements confirmed that the theoretical predictions were correct.
The researchers recently published their findings in Physical Review Letters.
A new benchmark for theory
Now that the theoretical and experimental data agree, it means that researchers using X-ray data from space telescopes can have greater confidence in the theoretical atomic models behind them.
Safronova said this new agreement between theory and experiment is particularly interesting as it makes it possible to study quantum electrodynamics (QED) — the study of how light and matter interact — when even more precise experimental data for 3C/3D transitions become available. This is because both theory and experiment must be very precise to see the subtle, but fundamental effects of QED in atoms and ions containing more than a few electrons.
The ability to perform very large-scale atomic computations also opens the door to testing other complicated fundamental physics questions that previously weren’t possible.
“Now that we know the computation is right, we can use it to guide experiments with other systems where we can now reliably predict atomic properties,” she said. “It also is a milestone that theory is trusted and our ability to compute things with quantum mechanics in complicated systems has reached a new level.”
Quantum mechanics is a fundamental physics theory that describes the behavior of matter and light at atomic and subatomic scales. It gives scientists the mathematical framework for computing properties of atoms to make precise theoretical predictions without an experiment.
Following postdoctoral studies, Cheung hopes to pursue a faculty position and inspire future theoretical physicists.
“Many students want to get their hands on things like lasers in the lab, but only a small percentage of people are interested in doing the theoretical calculations,” said Cheung. “I want to recruit more people interested in doing these types of calculations which will be monumental over the next decade for the new technologies and quantum sensors that are being developed now.”
Contact Us
Have a UDaily story idea?
Contact us at ocm@udel.edu
Members of the press
Contact us at 302-831-NEWS or visit the Media Relations website