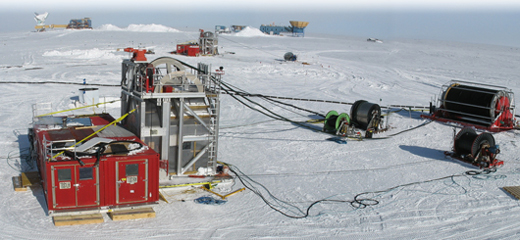
Cosmic Cool
Tiny neutrinos travel through space to South Pole telescope
RESEARCH | A huge telescope built deep into the crystal-clear ice of Antarctica has led to a discovery that received the Physics World “Breakthrough of the Year” award for 2013 and that scientists say ushers in a new age of astronomy.
The IceCube telescope was constructed over seven years in the harshest conditions on Earth by an international collaboration, with the support of the National Science Foundation, in which UD astronomers played a key role. Researchers using observations from the mile-deep instrument have now found for the first time some high-energy neutrinos of extraterrestrial origin—tiny particles that have no electric charge and are sometimes called “messengers of the universe.”


Neutrinos can zip right through your body, the walls of your house and entire planets, even emerging from near the surface of fascinating and frightening black holes.
“One of the interesting things about neutrinos is that they pass right through the Earth, and they’re neutral, so you don’t see them,” says Tom Gaisser, the Martin A. Pomerantz Chair of Physics and Astronomy at UD, who has worked with IceCube since its construction began in 2003. Because neutrinos are detected only in the infrequent cases where they interact with matter, “They’re rare and hard to study,” he says.
Gaisser leads a team of UD researchers who have been instrumental in building and maintaining the IceCube South Pole Neutrino Observatory’s surface array of detectors, known as “IceTop,” during the past decade. These detectors help scientists to rule out the interference caused by neutrinos produced locally in our atmosphere and to focus instead on more energetic particles produced light years away from Earth. It’s possible that those particles might even have been produced in the radiation field left over from the Big Bang, which most astronomers believe formed the universe.
Using the observatory, the IceCube collaboration recently observed 28 very high-energy particle events and determined that about a dozen of them likely came from outside our solar system. These observations constitute the first solid evidence of neutrinos coming from “cosmic accelerators”—potentially such sources as exploding stars or accreting black holes. The research was the cover story of the Nov. 22 edition of the journal Science and a few weeks later was cited as the year’s “top breakthrough.”
Gaisser says he was surprised by the designation, but only because he didn’t know that the British magazine Physics World gave such an annual award. He wasn’t surprised, however, that other scientists recognized the significance of IceCube’s observations.
“This is the first indication of very high-energy neutrinos coming from outside our solar system,” says Francis Halzen, principal investigator of IceCube from the University of Wisconsin–Madison. “This is the dawn of a new age of astronomy.”
In explaining its selection for the 2013 award, Physics World wrote: “IceCube can determine the direction from which a neutrino came, making it an incredibly useful telescope. Indeed, it could solve an important astrophysical mystery by revealing the origins of cosmic rays.”
According to Gaisser, the answer will depend on the number of neutrino events captured by IceCube, from which geometric calculations can be made to trace the high-energy neutrinos to specific astronomical sources.
Billions of neutrinos pass through every square inch of Earth every second, with the vast majority of these subatomic particles originating either in the sun or in Earth’s atmosphere. Far rarer are neutrinos from the outer reaches of our galaxy or beyond, which have long been theorized to provide insights into the powerful cosmic objects from which they originate: supernovae, black holes, pulsars, active galactic nuclei and other extreme extragalactic phenomena.
When neutrinos interact with matter—either in the rock or ice below IceCube or within the ice of the detector itself—the event creates a charged particle, which is detected as a flash of light and can then be analyzed.
“The first task of IceCube is to find the neutrinos from among the large number of cosmic ray events and then, having done that, to find the very few, very high-energy neutrinos [that come] from outside the solar system,” Gaisser says.
The analysis presented in Science reveals the first high-energy neutrino flux ever observed, a highly statistically significant signal that meets expectations for neutrinos originating in cosmic accelerators. The 28 high-energy events were found in data collected by IceCube from May 2010 to May 2012.
“This is a very exciting time for IceCube,” Gaisser says. “Having discovered some neutrinos from astrophysical sources, our job now is to discover what those sources are and where they’re coming from.”
IceCube: One extreme to the other
An enormous telescope buried under a mile of ice at the bottom of the globe may not seem the most obvious way to study the far reaches of the universe.
But scientists who investigate neutrinos know that the tiny, space-traveling particles are extremely hard to detect and that the most effective way to study them is to have them pass through a large amount of clear material, such as pristine water or ice. In that way, the ice—which is unusually clear and dense at the South Pole—can filter out other events and also allow neutrinos to react with hydrogen or oxygen in the water molecules to form charged subatomic particles that are easier to observe and analyze.
That’s what researchers were thinking about when they came up with the idea for IceCube, the collaborative project that took some 250 specialists from about 40 institutions seven years to build, with financial and logistical support from the National Science Foundation. In 2013, the project garnered widespread attention for making the first-ever observation of high-energy cosmic neutrinos.
“The ability to detect cosmic neutrinos is a remarkable achievement that gives astronomers a completely new way of studying the cosmos,” says Hamish Johnston, editor of physicsworld.com, which called the discovery the top breakthrough of the year. “The judges of the 2013 award were also impressed with the IceCube collaboration’s ability to build and operate a huge and extremely sensitive detector in the most remote and inhospitable place on Earth.”
IceCube is composed of 5,160 sensors called digital optical modules suspended like beads on a necklace along 86 cables embedded in a cubic kilometer of ice beneath the South Pole. The cables were installed in holes drilled into the ice using high-pressure, heated water. During the construction from 2003 to 2010, work could be done only during the Antarctic summer, from late October to early February.
Now, the detectors collect data year-round, with a large on-site computer farm that filters out irrelevant events and sends selected information by satellite to researchers working around the world.
Article by Tracey Bryant and Ann Manser, with additional information from the IceCube Collaboration, University of Wisconsin