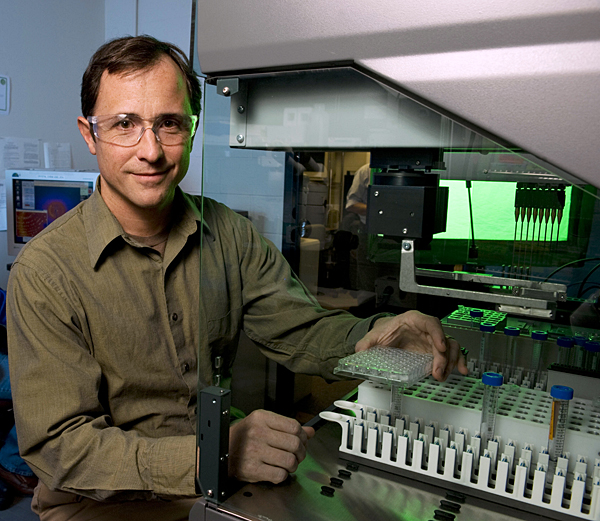
All the right moves
Subtle moves by proteins play important 'sparkplug' role
4:28 p.m., June 16, 2011--Just as a wink of the eye can trigger a chain reaction of events, U.S. researchers have revealed that subtle movements of proteins play an important role in sparking the complex chemical reactions that govern cell life.
The findings are reported in the June 13 issue of the Proceedings of the National Academy of Sciences by biochemist Brian Bahnson and graduate student Ming Dong from the University of Delaware and chemist Judith Klinman and graduate student Zachary Nagel from the University of California Berkeley.
Research Stories
Chronic wounds
Prof. Heck's legacy
Every cell produces enzymes -- the protein molecules that speed up chemical reactions to do all the things that cells need to do, from producing energy to building up biomolecules and complex cellular structures. Each enzymatic protein triggers a specific chemical reaction at a remarkable rate -- an elusive process that scientists are working to more fully understand.
One area of intensive research revolves around the role of protein dynamics in enzyme catalysis. Enzymes have unique 3-D structures that were once thought to be static, with precise arrangements of amino acids that dictated the protein’s role and function. But then scientists discovered that these proteins move.
Bahnson, an associate professor in the Department of Chemistry and Biochemistry at UD, was among the scientists who worked on such research in a mammalian system -- horse liver -- when he was a postdoctoral fellow in Klinman’s lab in California over a decade ago.
Recently, Klinman and Bahnson re-established their research collaboration and began examining the enzyme alcohol dehydrogenase from Bacillus stearothermophilus, a heat-resistant bacterium found in warm compost piles to hot springs and spoiled dairy products, to gain insight into how an enzymatic protein moves when it operates below its optimal temperature range.
“Proteins wiggle -- that’s just their nature,” Bahnson says. “When they are heated, they move even more. However, in cooling down the protein, we’ve found that there are much more subtle, evolved, ordered movements that also are critical to the process of catalysis.”
One of the consequences of these movements is referred to scientifically as “tunneling.”
“Imagine yourself a hiker coming up a mountain, and the elevation of that mountain is represented by energy,” Bahnson explains. “To get to the other side, you hike up to the lowest saddle point. That’s what happens in a classical reaction. But with certain enzyme systems, you only need to go halfway up the mountain because you can tunnel through it. Because you don’t need to hike up as high, you’re much faster at reaching the other side.”
The heat-loving Bacillus stearothermophilus studied by the researchers operates optimally at 70°C (158°F). The scientists cooled one of its enzyme down to a dynamic phase transition temperature of 30°C (86°F) and then characterized the enzyme across, above and below this 30°C threshold. They discovered trends in the protein’s dynamic properties and tunneling based on a non-linear relationship between the rate of the reaction versus temperature at a level never seen before. They reported a physical parameter known as the Arrhenius pre-factor at 1025, which was eight orders of magnitude larger than previously recorded, confirming that more complex factors are contributing to the catalytic reaction than just temperature.
“These subtle ordered motions, where the atoms are moving closer together as hydrogen prepares to be transferred in the reaction, are actually setting up the chemical reaction for tunneling. It’s a new concept,” Bahnson says.
Although large protein movements can be detected by X-ray crystallography, the more modest ordered movements the team uncovered are detectable primarily by kinetic data and the temperature dependence of the rates of reaction.
“This is basic research that helps us better understand how proteins work in their link to catalysis and can help inform the development of new enzyme catalysts,” Bahnson says. “The unique experimental data presented in our recent work will provide a framework for validating future computations that can give a clearer picture of how enzymes work.”
Article by Tracey Bryant
Photo by Kathy F. Atkinson